Chemistry | Solid-State Batteries: What Are They?
- Annabelle Bennett
- Apr 7
- 5 min read
Updated: 1 day ago
Batteries. The modern world depends on them for everything, from the cars we drive to the technology that powers our lives. From the very beginning, scientists and policy planners have recognised that a genuine shift to a green and renewable future hinges on a breakthrough in this field.
Undeterred, scientists and researchers have spent decades experimenting, refining, and pushing the boundaries to develop more efficient, sustainable, and safer battery technologies. And at long last, the field is beginning to yield results.
Solid-State Batteries: A Chemistry Defined By Electrolytes
At the forefront of the energy storage field are solid-state batteries, a new and promising alternative to the traditional lithium-ion format. By replacing liquid electrolytes with solid materials, these batteries not only redefine safety standards by eliminating risks like dendrites but also pave the way for higher energy densities and a reduced environmental impact.
However, solid-state batteries aren’t as straightforward as they might initially seem. There isn’t just one type of battery; there are several, each with fundamental distinctions, characterised by the solid-state electrolyte medium they use.
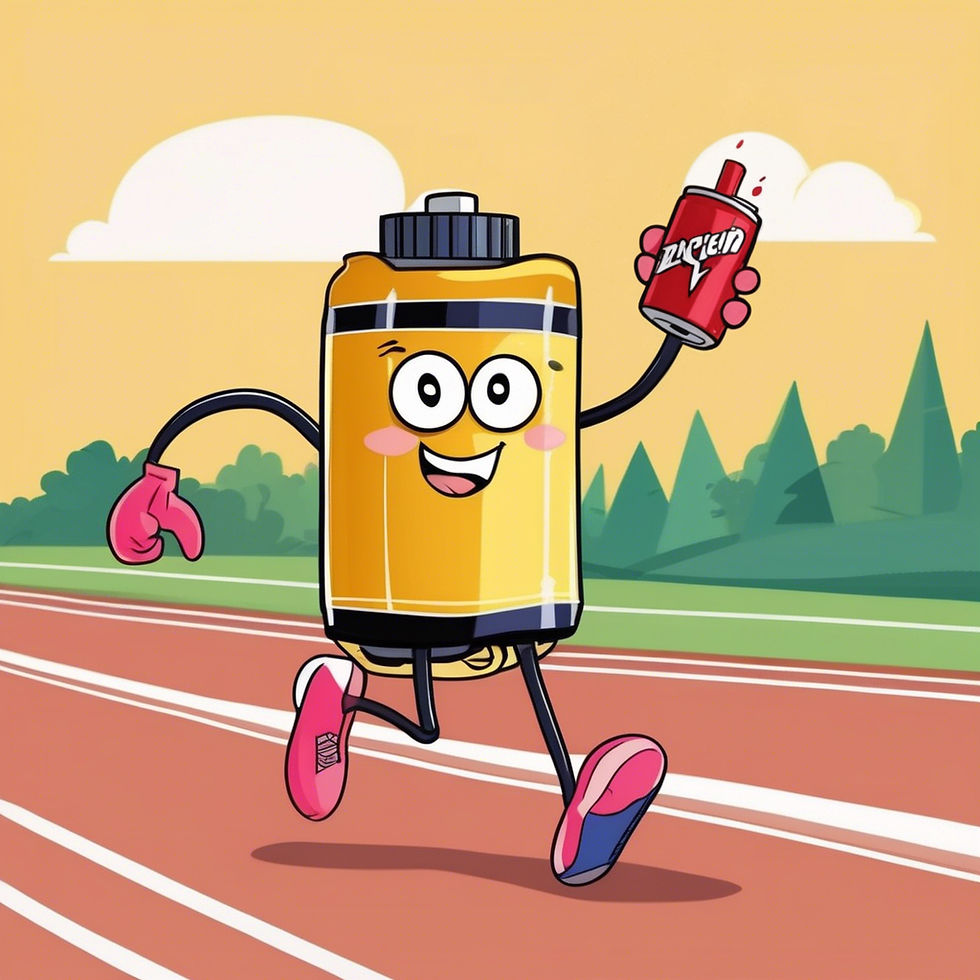
Inorganic Solid-State Batteries
Use inorganic materials, primarily ceramics, as the solid-state electrolyte. Specifically, lithium garnets, or lithium phosphorus oxynitride, which are highly valued for their exceptional ionic conductivity—a characteristic that translates into faster charging times and improved stability.
This system overcomes several drawbacks of liquid electrolytes, including leakage, flammability, and degradation over time, positioning solid-state alternatives as the ideal choice for EVs and portable electronics. With the global shift away from fossil-fueled engines and the constraints of current systems, this technology stands out as one of the few uniquely equipped to drive the transition and is expected to be phased into the market over the next decade.
What’s perhaps more significant is that oxide-based electrolytes are both stable and non-toxic, which makes them relatively eco-friendly. Their robust chemical structure also simplifies recycling, reducing reliance on new mining operations and helping to ensure sufficient supply for a full transition.
Organic Polymer Solid-State Batteries
Relying on polymer materials, such as polyethylene oxide, to function as the solid electrolyte, these polymers enable ion transport within the structure of their polymeric chains. The key advantage of this system lies in its remarkable structural flexibility, thinness, and lightweight design potential.
This has caught the attention of technology companies looking to harness it for the next phase of digital augmentation—wearable electronics. Not merely in the form of a smartwatch but, quite literally, transforming your clothes into a computer.
Organic polymer batteries are also easier and relatively cheaper to manufacture than their inorganic counterparts. However, they do come with certain drawbacks: their conductivity is comparatively low, which makes them unsuitable for meeting industrial demands. Their complex chemical composition also poses significant challenges when it comes to recycling.
Hybrid Solid-State Batteries
By combining the properties of inorganic and organic systems, this composite material achieves a balance of ionic conductivity, stability, and mechanical flexibility. It also addresses the challenges associated with maintaining a stable interface between electrodes and electrolytes. In conventional solid-state batteries, these interfaces often encounter issues such as poor contact, high interfacial resistance, and chemical instability, all of which hinder ion transport and reduce battery efficiency.
As for its specific applications, while the system is versatile enough to be used in both EVs and wearable electronics, it is more likely to find a home in emerging technologies such as robotics or flexible displays, where adaptability and versatility are crucial.
The environmental impact of this material is less clear-cut and largely depends on the specific combination of materials used. However, its mixed composition can make recyclability significantly more challenging compared to alternative systems.
The Future of Solid-State Battery Technology
Although solid-state batteries are expected to become a cornerstone of technology and energy use in the future, manufacturing remains a key challenge—one without a short-term solution, as it requires industrial complexes that can take several years to establish. While a few companies are ahead in the field, such as Honda, which demonstrated a production line for all-solid-state batteries in 2024, there still aren’t enough facilities being built to meet the anticipated demand.
The very same problem arises when it comes to recycling. Although solid-state batteries are far more sustainable and recyclable, the facilities required to harness these advantages aren’t yet in place. Consequently, material sourcing for the industry remains a challenge—one that is likely to worsen due to the current economic slowdown and the demise of globalisation.
Another concern being raised by campaigners is the issue of rapid redundancy. Current EV models could quickly lose economic value and, as a result, be scrapped prematurely, thereby negating the carbon offsets they were designed to achieve. The same risk applies to early iterations of solid-state battery EVs, which may be rendered obsolete by rapid advancements in the technology. In the short to medium term, this could mean that the environmental benefits of the technology might not materialise.
The TLDR
Solid-state battery technology has the potential to revolutionise global energy use, with a broad range of systems tailored to specific niches. Whether this will materialise into a market-wide rollout within the next decade remains to be seen.
While manufacturing and recycling centres are being established, the recent economic slowdown and the shift away from globalisation are likely to stall progress. That, combined with the prospect of rapid redundancy, may undermine sustainability efforts aimed at tackling global warming—an issue that appears to have been sidelined by much of the global policymaking community.
So, a cautiously pessimistic outlook might be the most realistic way to view the potential of this technology.
Comments