Biology | Epigenetic Memory: The Foundation Of A Biotechnological Revolution
- Phillip Drane
- Feb 25
- 6 min read
Updated: 3 days ago
Epigenetic memory has, for some time, been a buzzword in an often overly politicised culture war that either misunderstands the science or denies it entirely. While it is true that it remains an emerging, evolving, and somewhat underdeveloped field of research, it is one supported by the wider scientific community and, more importantly, by data.
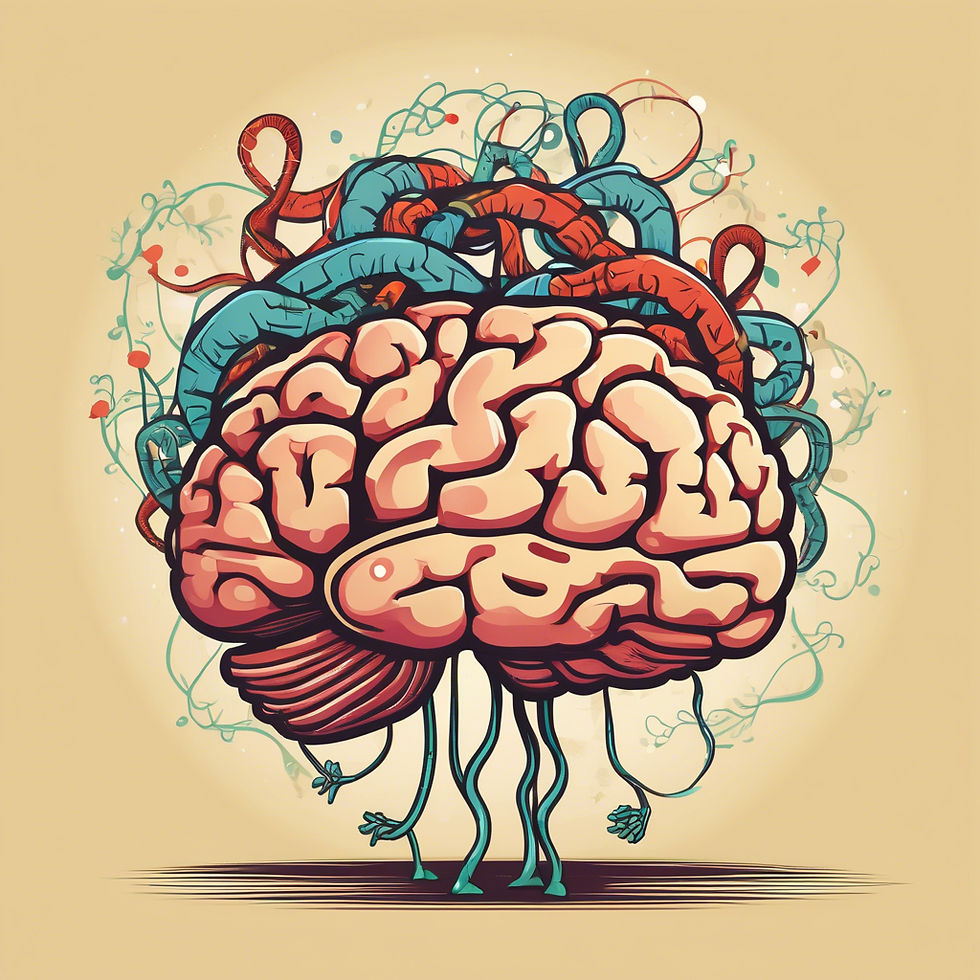
The Mechanics Of Epigenetic Memory
The term was coined by Conrad Waddington, a British embryologist, who used the phrase to describe the interactions between genes and their products. In its modern context, it loosely refers to aspects outside of the traditional mechanisms of inheritance – the idea that environmental factors cause changes in gene expression that are then passed down through generations without altering the underlying DNA sequence.
Non-traditional mechanisms include DNA methylation, histone modification, and non-coding RNA molecules. These epigenetic modifications can silence or activate specific genes, influencing an organism’s traits and behaviours. In essence, it's how our experiences and environment can leave a lasting mark on our genes, influencing how they are expressed in both us and our descendants.
The research being conducted in this field is primarily performed by exposing organisms to various environmental conditions and tracking the resulting epigenetic changes across generations. How exactly these modifications are subsequently inherited and maintained is examined in three main ways: the study of DNA methylation patterns via bisulfite sequencing, analysis of histone modifications with ChIP-sequencing, and examining the roles of non-coding RNAs through RNA sequencing.
Epigenetic Memory: Increasing The Size Of The CRISPR Toolbox
Although preliminary exploration into the field has provided insights into disease, adaptation, and heredity, it is only very recently, with the advent of AI and the modification of CRISPR technology, that this science could take the lightyear jump needed to make it revolutionary.
Understanding how these mechanisms work is one thing; it is another thing entirely to leverage them to produce a desired outcome. Researchers have begun to address this with Chem-CRISPR/dCas9. Although still on a long road to full development, the technique could allow a relatively quick and easy way to turn certain genes on or off.
Now, that sounds a lot like traditional Cas9 CRISPR, so you may be wondering what exactly the difference between the two is.
Well, regular CRISPR/Cas9 is a gene-editing tool that introduces double-strand breaks in DNA at specific locations. The Cas9 protein, guided by a single RNA, cuts the DNA, and the cell’s natural repair mechanism fixes the break, resulting in a gene modification. The technology is used for gene insertion, knockout, and general genetic modification with the goal of understanding gene function and developing gene therapies.
Chem-CRISPR/dCas9 is a similar but altogether different beast that uses a deactivated Cas9 protein, which lacks the ability to cut DNA, but is instead fused with either chemical inhibitors or epigenetic modifiers. These target specific genome locations, allowing for the modulation of gene expression without altering the DNA sequence, importantly this is a reversible process. So, an easy way to think about it is: traditional CRISPR is for permanent changes to the DNA sequence, whereas Chem-CRISPR/dCas9 is for precise and reversible control of gene expression.
Thinking about the differentiation of techniques in a practical scenario, imagine we reach the stage where we know how to activate the gene that causes teeth to regrow. Traditional CRISPR/Cas9 might not be the ideal choice, as it would basically give an individual hyperdontia—a condition where teeth continuously grow and fall out. In contrast, Chem-CRISPR/dCas9 could turn the gene on, giving an individual a new set of teeth, and then off once they have fully grown.
Landmark Studies, Discoveries, & Evidence
Experimental models in this field of research have ranged from simple organisms like nematode worms and fruit flies to more complex systems like mice and human cell cultures. Generally speaking, there have been three landmark studies that have acted as stepping stones for development in the field.
The first was a study conducted by the European Molecular Biology Organisation in 2017, which demonstrated that environmental genetic changes can create a mark on genetic expression. In the case of roundworms, the experimental model, this mark persisted for 14 generations, which, at the time, was the longest span of environmentally induced genetic change observed in an animal. In other words, it was one of the first studies that demonstrated transgenerational epigenetic inheritance.
The second study, conducted by researchers at the University of California in 2022, built on this. It focused on a particular modification of a histone protein (H3K27me3). The epigenetic mark H3K27me3 references the methylation of a particular amino acid, leading to DNA being less packed and genes in that region being less accessible for activation.
Once again using roundworms as the experimental model, the study established a cause-and-effect relationship between sperm-transmitted histone marks, gene expression, and development in offspring and grand-offspring.
The third landmark study, conducted by École Polytechnique Fédérale de Lausanne (EPFL) in 2024, demonstrated the role of epigenetic state in neurons, showing that epigenetic changes are crucial for memory formation and learning.
Researchers in the study focused on the flexibility of the chromatin – the packaged form of DNA inside a cell. They discovered that the epigenetic state of a neuron, specifically the openness or compactness of its chromatin, plays a determining role in which neurons are involved in forming a specific memory.
Using mice as the experimental model, researchers used a virus to deliver epigenetic enzymes that artificially induce the openness of the neurons' chromatin. The study found that mice with more open chromatin states in their neurons learnt better. When the opposite approach was taken – researchers generating a compactness of chromatin – they found that the mice’s ability to learn was impaired.
The study is particularly significant as it shifts the focus from the traditional view of synaptic plasticity to epigenetic changes in the nucleus of the neurons, which could fundamentally alter the way in which cognitive disorders are treated and understood.
Where Will The Biggest Changes From This Technology Be Felt In The Next Five Years?
Looking forward, there are two key areas where you are likely to see significant changes as a result of this technology. The first is in the medical field, where you will start to see targeted therapies that create a personalised approach tailored to your epigenetic profile. It is also very likely that the research conducted on the epigenetic state in neurons in relation to memory will build out further, potentially resulting in a silver bullet for neurodegenerative diseases like Alzheimer's and Parkinson's.
The second area is agriculture, where epigenetic research is going to be harnessed to speed up the development of crop varieties that have enhanced resistance to the stresses of modern climate change. This same technology can also increase the yield and nutritional content as arable land decreases and the population rises.
Comments