AI | Immortality: Could It Be Achieved By Nanites?
- Phillip Drane
- 5 days ago
- 10 min read
Updated: 2 days ago
Nanites, short for "nanorobots" or "nanomachines," are microscopic robots built at the nanometre scale—one-billionth of a metre. The concept's roots trace back to the field of nanotechnology, largely pioneered by Richard Feynman, who introduced the idea of manipulating matter at the atomic scale during a lecture in 1959.
However, it was science fiction that propelled the idea into popular consciousness, with zeitgeist shows like Star Trek showcasing the medical uses of this technology—applications that, remarkably, are now edging closer to reality.
Being smaller than a single human cell, nanites could theoretically interact with biological systems on a molecular level, provided they are made from non-reactive, biocompatible materials such as carbon nanotubes, metals, or even biological components.
In the future, medical nanites could navigate the bloodstream, repairing damaged cells, eliminating pathogens, and even reversing the effects of ageing at both the cellular and tissue level—meaning no more wrinkles.
But beyond this, at the very edge of nanotechnology's visible potential, lies the holy grail of immortality. One day, nanotechnology could evolve into a system of perpetual repair. Yes, you could still fall victim to a falling piano, but natural death—or even ageing—would be firmly off the cards.
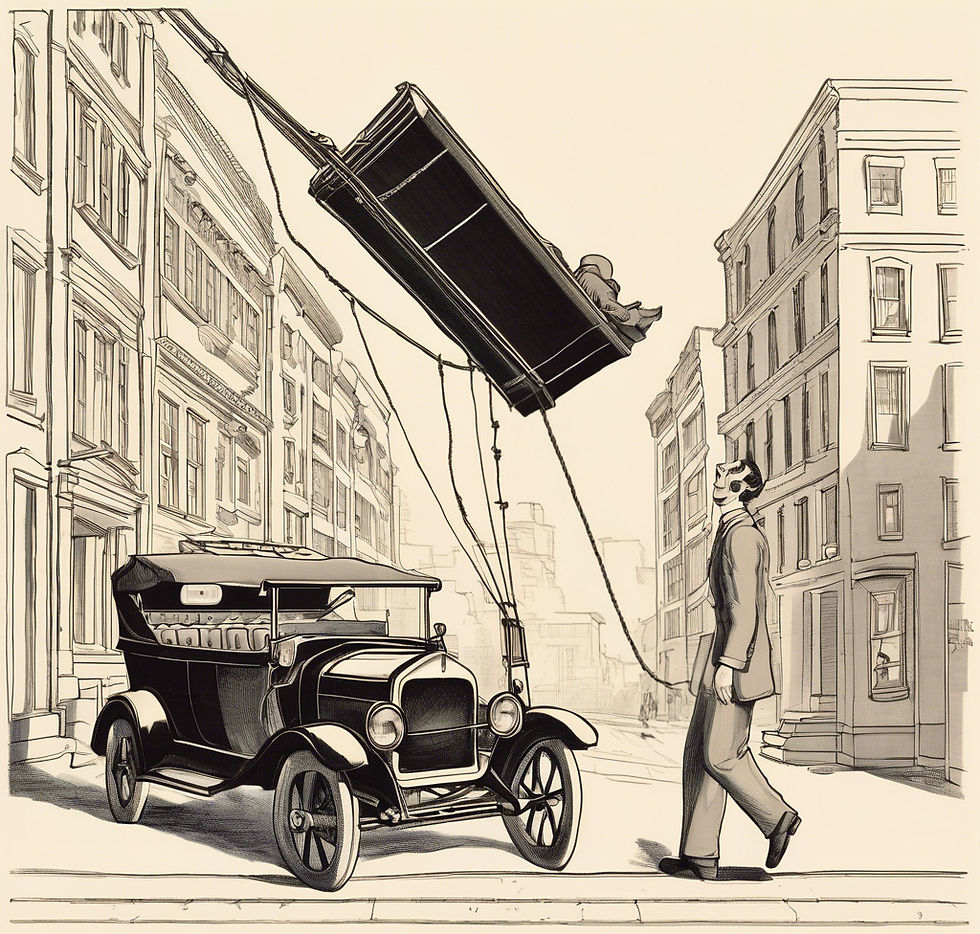
Medical Nanites: Theoretical Mechanics & The Role Of AI Programming
The operation of medical nanites centres on their ability to interact precisely with their surrounding environment at a microscopic scale.
In a hypothetical scenario, nanites would need to be equipped with tiny sensors to collect data and detect specific biological or chemical markers, enabling them to identify diseased or damaged cells within the body. Upon detection, nanoscale actuators and manipulators could perform intricate tasks, such as repairing damaged DNA, eliminating harmful pathogens, restoring cell functions, delivering therapeutic agents directly to targeted sites, and interacting with key cellular components.
Isolated nanites operating in the body wouldn’t be enough—instead, millions (or even hundreds of millions) would need to work in unison. Functioning as a coordinated swarm, these tiny machines would share real-time sensor data and manage tasks much like a biological network. Each nanite, guided by a miniature onboard AI, would make autonomous decisions using advanced algorithms to predict and address issues before they arise—ensuring proactive maintenance of the body’s health. Over time, as this adaptive system learns from an individual’s unique biology, it could evolve into a highly personalised medical tool.
What Does The Current Field Of Nanite Technology Look Like?
Although AI programming is on track to reach the foundational threshold required for this technology to advance, miniaturising these systems to fit within nanites while maintaining their computational capabilities remains a daunting task. It is also an ethically challenging endeavour, particularly in the absence of the necessary regulatory frameworks. Even so, recent years have seen significant strides that hint at the potential of overcoming these obstacles.
Nanoparticles are already being utilised in targeted drug delivery systems, transporting medication directly to specific cells. This technique has demonstrated significant potential in cancer treatments, where precise targeting of cells leads to improved clinical outcomes and noticeably fewer side effects compared to traditional methods such as chemotherapy.
Carbon-based materials, such as hydrogen-substituted graphdiyne, have been utilised to create artificial muscles that mimic natural movement, exhibiting precise, rapid, and continuous deformation capabilities. This innovation, inspired by the structure of a butterfly's proboscis, offers a promising foundation for the development of nanites capable of performing precise mechanical tasks at a molecular level.
New nanosensor technology promises real-time patient monitoring and early disease detection by tracking specific biomarkers in the body. A 2025 academic paper explored the benefits of combining this technology with the CRISPR/Cas system, creating ultra-sensitive and precise detectors capable of identifying cancer-related nucleic acids. Researchers also found that different nanomaterial combinations boost detection sensitivity and specificity—a breakthrough that could soon lead to next-generation nanites with advanced health monitoring capabilities.
Self-healing materials are another technology that has recently seen breakthroughs. These materials utilise embedded nanoparticles or microcapsules filled with a healing agent that, when damage, such as cracks, occurs, release their contents to bond with the surrounding material and seal the breach. Researchers have suggested that advancements in this field could eventually lead to nanite systems designed to repair damaged tissues and materials.
However, despite the promise and progress in the field, several key hurdles must still be overcome before the science can be said to have crossed the Rubicon.
The Nanomaterial Engineering Issue
Nanomaterial engineering involves developing materials and components that operate reliably at the nanoscale. Currently, researchers are exploring innovative solutions, such as carbon-based nanomaterials and biocompatible designs. Although there are several promising candidates, these are likely to be a decade or more away from reaching the mainstream market, as the necessary manufacturing techniques and facilities are not yet in place.
Perhaps more importantly, achieving the precision and durability required for medical applications remains a complex endeavour. Researchers must overcome issues such as toxicity, inconsistent characterisation techniques during both the development and analysis of potential materials, and a limited understanding of the potential impact on cellular energy homeostasis and immune response.
Nanites: The Energy Issue
Powering nanites within the human body presents another challenge, as traditional energy sources are impractical at this scale. There are a number of different theories regarding how exactly this could be achieved; however, perhaps the most promising approach—backed by the most real‐world testing—involves triboelectric nanogenerators (TENGs) and piezoelectric nanogenerators (PENGs). It is worth clarifying that these technologies are still in the early stages of development.
TENGs generate energy through the triboelectric effect, whereby the contact and separation of different materials results in the transfer of electrons and the production of an electric charge. By employing nanoscale thin films or patterned surfaces, TENGs can maximise charge generation by enhancing the surface area, efficiently harvesting mechanical energy from subtle movements within the human body, such as blood flow or cellular vibrations.
PENGs, on the other hand, rely on the piezoelectric effect, in which certain materials generate electricity in response to mechanical stress, such as bending or compression. At the nanoscale, materials like zinc oxide nanowires or thin piezoelectric films are used to capture energy from body movements, such as muscle contractions or even cellular activity. These systems could enable nanites to autonomously harvest energy, allowing them to perform tasks like cellular repair, drug delivery, or diagnostics without relying on external power sources or bulky batteries.
The Issue Of Nanite Communication
Nanites require effective communication methods to coordinate tasks and interact with external systems, and two key approaches are being explored: electromagnetic signals and chemical messengers. Electromagnetic communication involves equipping nanites with miniature antennas or receivers to emit and detect signals, such as radio or infrared waves. This capability allows nanites to share information about their locations, tasks and environmental conditions, enabling synchronized actions and swarm intelligence. However, designing antennas that are small enough to function efficiently at the nanoscale is a complex challenge, and electromagnetic interference in biological environments could disrupt communication.
Chemical messengers, inspired by biological systems, offer another viable method. Nanites could release specific molecules into their environment, which other nanites would detect using nanosensors. These molecules could encode messages through their composition, concentration, or release pattern, allowing nanites to share instructions or alerts. This approach is particularly well-suited for biological environments, where chemical signalling occurs naturally. The challenge lies in designing nanites to produce, release and detect these chemicals with high precision, as well as ensuring scalability for larger systems.
Hybrid systems that combine electromagnetic signals and chemical messengers are also under consideration, leveraging the strengths of both methods. Electromagnetic signals could handle long-range communication, while chemical messengers might be better suited to local interactions. Further research is needed to address challenges such as energy efficiency, scalability and reliability in these communication methodologies.
The Issue Of Robust Fail-Safes
Ensuring the safe operation of nanites within biological environments demands robust safeguards. Fail-safe systems are essential for preventing malfunctions or unintended consequences during operation. Equally important is the integration of self-destruction mechanisms: these allow nanites to be safely deactivated or dismantled once they have completed their tasks or if they pose a risk.
Several approaches to this challenge are being researched, such as chemical triggers that dissolve nanite components when exposed to specific conditions, and thermal deactivation using heat-sensitive materials. External signals, like electromagnetic pulses, could also initiate self-destruction in a controlled manner.
Redundancy in these systems is vitally important. Multiple layers of fail-safe protocols must be in place to ensure stability if one or more systems fail. Isolation protocols that enable malfunctioning nanites to temporarily disengage from their surroundings are also crucial in minimising potential harm or a cascading effect.
Development of these systems remains a complex task, as many of the required technologies are not yet available—and even when they are, a decade of lab testing may be necessary before any human trials can begin.
The Wider Potential Of Nanites: A World Transformed
Yes, the potential of this technology in the medical field could produce a leap like none we have ever seen—one that might fundamentally redefine humanity and society—but it is not the only use.
Nanite technology could have profound implications for environmental sustainability and the fight against pollution at the molecular level. By breaking down harmful substances into benign components and removing plastics from our oceans, the technology could pave the way for innovative clean-up strategies. Early iterations have already shown promising results, and broader adoption is anticipated in the coming years.
In agriculture, nanites could enhance crop resilience by delivering nutrients directly to plant cells or by protecting them from pests without resorting to harmful chemicals. Global industry and manufacturing could also benefit, as self-replicating nanites might enable rapid, precise production at an atomic scale, leading to the creation of materials and devices with unprecedented efficiency. The use of the technology could even extend into space exploration, where they might perform repairs on spacecraft or assist in constructing habitats in inhospitable environments.
The TLDR: Could Immortality Be Achieved By Nanites?
Experts estimate that we are still decades away from realising the full potential of nanites. However, incremental progress in nanotechnology and artificial intelligence suggests we may see early prototypes or limited applications—such as targeted drug delivery or diagnostic tools—within the next 10 to 20 years. The journey towards fully autonomous, multifunctional nanites will likely span several decades, driven by interdisciplinary collaboration and sustained innovation.
The societal impact of nanites could be profound, offering the potential to address inequalities in healthcare and resource management. However, such transformative technology also raises ethical challenges, including concerns about access, control, and unintended consequences. With responsible development, nanites could redefine humanity's relationship with technology, enabling not only a future of endless possibilities but also one in which you could theoretically live forever.
Comments